Publications
Teratology Primer, 3rd Edition
What are the Possible Consequences of Pre-Conception Male Germ Cell Exposures on Fertility and Progeny Outcome?
Bernard Robaire and Barbara F. Hales
McGill University, Montreal, Quebec, Canada
A father’s age, occupation, life style and exposures may affect both his fertility and the health of his offspring. There is evidence from animal models that some chemicals, and even viruses that are present in seminal fluid, affect progeny outcome. Data from both human and animal studies reveal that paternal exposures may have adverse effects on male germ cells, decreasing germ cell numbers, and/or altering germ cell integrity. These effects may lead to outcomes that include infertility, pregnancy loss or spontaneous abortion and birth defects, or effects that are manifested only later in life, such as childhood cancer, behavioral effects or learning deficits and metabolic syndrome. We also now have evidence that some paternally-mediated adverse effects on progeny may be transmitted to subsequent generations.
Male Germ Cell Production
Male germ cells are “engineered” to provide the paternal genome to the conceptus. Spermatogenesis, the process by which male germ cells develop, is highly ordered and occurs at an astonishing rate. Indeed, in humans approximately 1,000 spermatozoa (mature male germ cells) are produced with every heartbeat; this amounts to about 100 million daily. Spermatozoa originate from a pool of germ stem cells that lie at the base of the seminiferous tubules in the testis. During spermatogenesis, these cells undergo several mitotic and two meiotic divisions, followed by a series of structural changes that include the creation of a tail that will be used for swimming, the shedding of most of the cell’s cytoplasm, the formation of an acrosome, a structure that will permit recognition and interaction with the oocyte and its surrounding element, and chromatin remodeling to repackage the haploid genome (Figure 1). This “repackaging” includes a change in shape, compaction of chromatin, modifications in protein expression, and alterations in metabolic activity. Extensive changes are observed in epigenetic marks, including alterations in sperm DNA methylation, histones and their modifications, and RNAs. The methylation of DNA at cytosine residues is the most studied epigenetic mark, but both histone modifications and the inclusion of a range of non-coding RNAs are also important. Histones are largely replaced by protamines in the final stages of spermatogenesis, allowing the DNA to be sufficiently condensed to fit in the sperm head. However, a proportion of the histones, ranging from 1-15% depending on the mammalian species studied, are retained. The histones and RNAs that are retained in sperm may serve as epigenetic marks that are transmitted to the oocyte at fertilization and are involved in the regulation of gene expression in early embryos.
Figure 1. Spermatogenesis in man. The formation of spermatozoa from spermatogonial stem cells is divided into three stages: 1-Proliferation, renewal and differentiation of spermatogonia (dark type A spermatogonia (Ad), pale type spermatogonia (Ap) and differentiated spermatogonia (B). The spermatogonia Ap and B divide by mitosis (M). All type B cells give rise to preleptotene spermatocytes PL). 2-Meiosis or reductional divisions involving primary and secondary spermatocytes. The prophase of the first meiotic division of primary spermatocytes go through the following steps: preleptotene (Pl) → leptotene (L) → zygotene (Z) → early pachytene (EP) → mid pachytene (MP) → late pachytene (LP). The late pachytene cells undergo the first meiotic division that gives rise to secondary spermatocytes (II), which soon undergo the second meiotic divisions that yield haploid spermatids. 3-Spermiogenesis or the metamorphosis of spermatids into spermatozoa. The following structures are labelled: Golgi apparatus (G), acrosome (A), centrioles (C), tail (T) and residual body (RB). Adapted from Clermont, Lalli, and Bencsath-Makkai, Light-Microscopic Histology Atlas. http://audilab.bmed.mcgill.ca/HA/
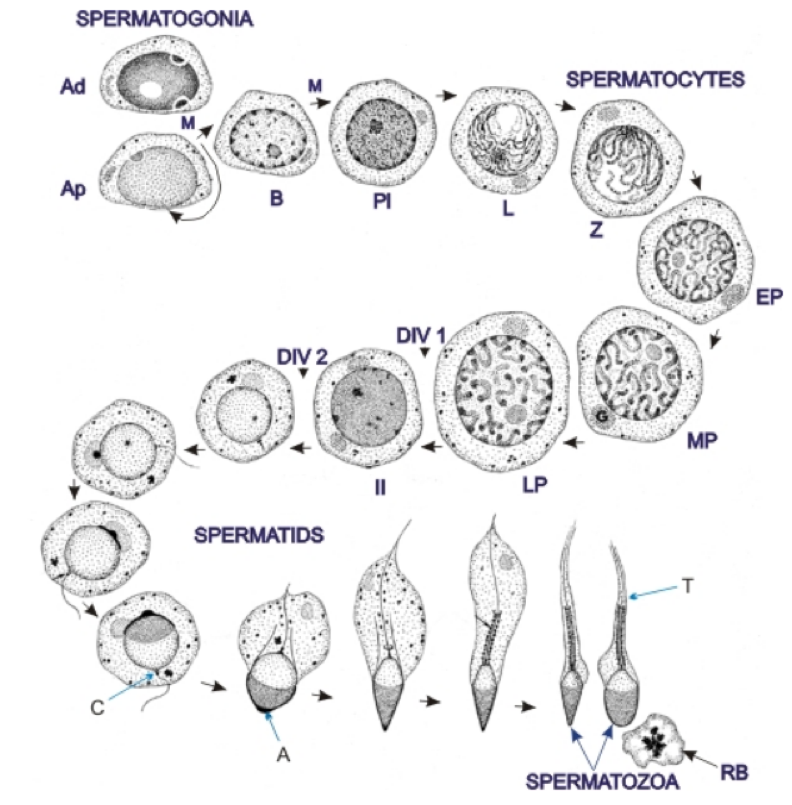
During spermatogenesis, germ cells progress from the base to the apex of the seminiferous tubules and are then released into the luminal fluid as spermatozoa. These spermatozoa leave the testis to transit through the efferent ducts and then the epididymis, where they acquire the potential for motility and the ability to fertilize an oocyte. The temporal events whereby undifferentiated spermatogonial germ cells develop into mature spermatozoa requires weeks (9 in man), is very complex, and is under tight hormonal control. These processes may be disturbed, damaged, or arrested by exposure to environmental chemicals, drugs, or altered ambient conditions.
Pre-Conception Male Germ Cell Exposures: Effects on Germ Cell Numbers or Quality
The adverse effects of various exposures or conditions on male germ cells may be manifested as a decrease in germ cell numbers, as a result of blocking mitosis and/or meiosis or of increasing cell death by inducing apoptosis. In 1992, Carlsen and coworkers triggered worldwide debate over a possible decline in sperm production and male fertility. A recent systematic review also reported a significant decline in sperm counts; between 1973 and 2011 this decline was 50-60% among men from North America, Europe, Australia, and New Zealand.
Exposure to xenobiotic chemicals may also affect germ cell quality, e.g., sperm motility or chromatin integrity, in the absence of an effect on sperm counts. Toxicants that affect sperm motility may decrease their ability to swim and fertilize an oocyte. Other parameters of germ cell quality that may result in male infertility include effects on sperm DNA integrity, chromatin structure and compaction, and the proteins associated with DNA. Using assays to assess these endpoints, it has been shown that exposure not only to physical agents and chemicals but also to lifestyle factors, such as stress, cigarette smoking, or a high fat diet, may affect the integrity of sperm chromatin, inducing structural, genetic and/or epigenetic abnormalities.
Pre-Conception Male Germ Cell Exposures: Effects on Fertility or Progeny Outcome
Toxicants may decrease male fertility. Alternatively, since damaged sperm may retain the ability to fertilize, they may induce post-fertilization adverse effects on progeny outcome, with consequences for both the immediate progeny and future generations (Figure 2). It is challenging to link specific human paternal exposures to adverse effects on pregnancy outcome. Infertility and pregnancy loss occur quite commonly in humans so the extent to which an exposure increases their incidence above background may be difficult to decipher. Other developmental outcomes of concern may be rare or are observed only after a long delay, making it necessary to conduct longitudinal studies in a large population of exposed individuals. Finally, paternal and maternal exposures frequently overlap so it may be difficult to ascertain the relative contributions of each parent. Notwithstanding these limitations, a number of epidemiological studies have reported associations between human paternal exposures and male infertility or abnormal progeny outcomes. An increase in time to pregnancy or a decreased fecundability odds ratio, an increase in spontaneous abortion/embryo or fetal loss, birth defects, childhood cancer, and behavioral effects or metabolic syndrome are all endpoints that have been used to assess the association of pre-conception paternal chemical exposures with adverse effects on fertility or progeny outcome. Many studies have focused on the consequences of occupational exposures – e.g. to pesticides, heavy metals, or organic solvents. Others have examined the effects of therapeutic exposures – e.g. to anticancer drugs or radiotherapy. Paternal exposures to radiation, solvents, heavy metals (e.g. mercury), pesticides, and hydrocarbons have been associated with an increased incidence of spontaneous abortion or miscarriage and birth defects. Still other studies report on the effects of environmental chemicals or lifestyle exposures – e.g. to phthalates (widely used as plasticizers) or components of cigarette smoke. For example, the exposure of male partners to certain phthalates (monomethyl-, mono-butyl, and monobenzyl phthalates) was more often associated with diminished couple fecundity than the exposure of female partners. Recently, studies have also examined the impact of paternal obesity and diet on progeny outcome. Obese men were found to have an increased percentage of sperm with abnormal morphology and DNA fragmentation and to be more likely to experience infertility and a reduced rate of live births per cycle of assisted reproduction technology than lean men.
Figure 2. A diagrammatic representation of the possible impact of paternal pre-conception exposures on male germ cells on fertility and progeny outcome.
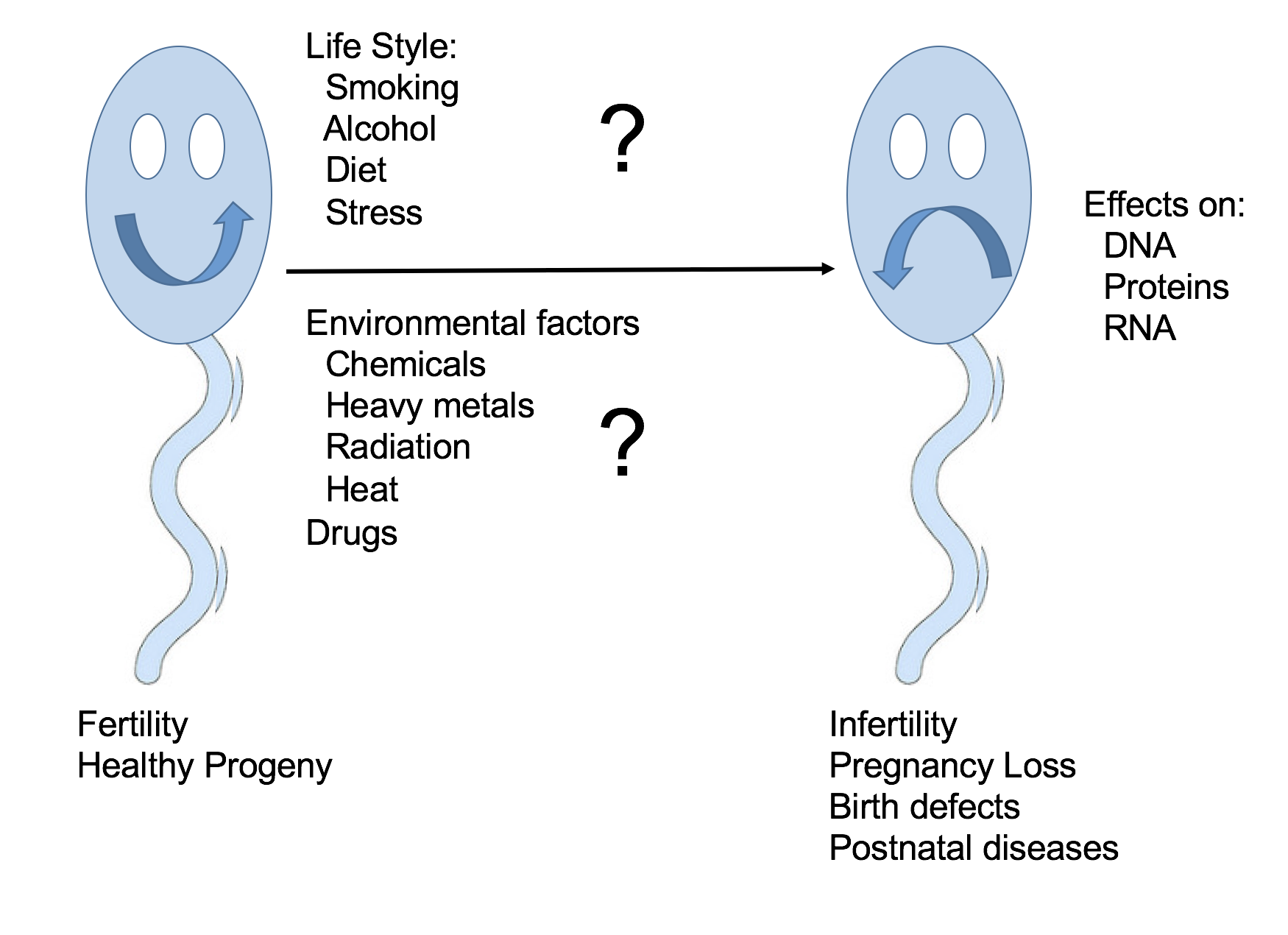
Animal models have provided a wealth of data on the impact of paternal exposures on male germ cells and progeny outcome. There has been an explosion of studies demonstrating that the mechanisms by which paternal exposures trigger developmental toxicity are highly varied. DNA damage may be responsible for the transmission to progeny of the effects of some paternal exposures. For example, a dose-dependent increase in heritable germline mutations at a minisatellite locus was observed in the children of men who smoke. A father's smoking prior to conception has also been reported to significantly predict DNA damage detected in the cord blood of their offspring.
We now know that the male germ cell transmits much more than its genome, as specified by DNA sequences, to the conceptus. Sperm methylation patterns have been reported to be altered by exposure to anticancer drugs, pesticides, particulate air pollution, and a high fat diet. The transmission of alterations in DNA methylation to offspring has been reported in some animal studies. Experiments with mice that overexpress a histone demethylase (KDM1A) in male germ cells have shown that altering histone modifications in spermatozoa can lead to developmental abnormalities in progeny that may be transmitted to subsequent generations. Paternal folate deficiency, resulting in altered DNA methylation, is associated with increased birth defects in the offspring. Sperm histone contents may also be altered by paternal diet. In an animal model, spermatozoa from males fed a high fat diet had an increased retention of histone H3 and a modification associated with active genes, the H3K4me1 mark, at promoter regions enriched with genes involved in embryonic development.
Mature mammalian sperm retain a complement of RNAs that can be transmitted to the zygote at the time of fertilization. It has been suggested that the RNA retained in sperm may contain specific transcripts that are crucial for embryonic development. Thus, a paternal exposure that leads to changes in sperm RNA content may affect embryogenesis. Paternal stress can alter the complement of sperm miRNAs and affect early chromatin remodelling events in the zygote, leading to a blunted stress phenotype in offspring. A paternal high fat and low protein diet may also alter the expression of sperm tRNA derived small RNAs (tsRNAs) that have been shown to be involved in paternally-mediated intergenerational inheritance. When the tsRNAs from rats fed a high fat diet were injected into control zygotes, the resulting progeny did not share the obese phenotype of their fathers but did show impaired glucose absorption. This phenotype was also observed in progeny obtained by the natural mating of male rats fed a fat diet. Thus, the exposure of male germ cells to a toxicant, or lifestyle factors, such as diet, may leave a legacy that has an impact not only on individual embryos but also on future generations.
Conclusions
There is abundant evidence that male-mediated adverse progeny outcome is a real phenomenon; both genetic and epigenetic mechanisms have been suggested. Should we be concerned about progeny outcome after the exposure of men to lifestyle factors or to chemical or physical agents? It is clear from human studies that environmental exposures may have an effect on fertility and increase germline mutations. More research is needed to investigate the association with specific birth defects and the underlying mechanisms.
Suggested Reading
Buck Louis GM, Sundaram R, Sweeney AM, Schisterman EF, Maisog J, Kannan K. Urinary bisphenol A, phthalates, and couple fecundity: the Longitudinal Investigation of Fertility and the Environment (LIFE) Study. Fertil Steril. 2014; 101(5):1359-66.
Carlsen E, Giwercman A, Keiding N, Skakkebaek NE. Evidence for decreasing quality of semen during past 50 years. BMJ. 1992; 305(6854):609-13.
Clermont Y. Kinetics of spermatogenesis in mammals: seminiferous epithelium cycle and spermatogenic renewal. Physiol Rev. 1972; 52:198–236.
Delbès G, Hales BF, Robaire B. Toxicants and human sperm chromatin integrity. Mol Hum Reprod. 2010; 16:14–22.
Downey AM, Robaire B, Hales BF. Paternally Mediated Developmental Toxicity. In: McQueen CA, ed. Comprehensive Toxicology, 3rd ed. Oxford: Elsevier, 2018. Vol. 5, pp. 100-117.
Hales BF, Robaire B. The Male Germ Cell as a Target for Toxicants. In: McQueen CA, ed. Comprehensive Toxicology, 3rd ed. Oxford: Elsevier, 2018. Vol 4, pp. 82-95.
Jenkins TG, Aston KI, James ER, Carrell DT. Sperm epigenetics in the study of male fertility, offspring health, and potential clinical applications. Syst Biol Reprod Med. 2017; 63(2):69-76.
Laubenthal, J., Zlobinskaya, O., Poterlowicz, K., et al. Cigarette smoke-induced transgenerational alterations in genome stability in cord blood of human F1 offspring. The FASEB Journal. 2012 26:3946-3956.
Levine H, Jørgensen N, Martino-Andrade A, Mendiola J, Weksler-Derri D, et al. (2017) Temporal trends in sperm count: a systematic review and meta-regression analysis. Human Reproduction Update. https://doi.org/10.1093/humupd/dmx022.
Zini A, Albert O, Robaire B. Assessing sperm chromatin and DNA damage: clinical importance and development of standards. Andrology. 2014; 2(3):322-5.